Journal of Creation 34(2):117–123, August 2020
Browse our latest digital issue Subscribe
The volcanism and age of Io
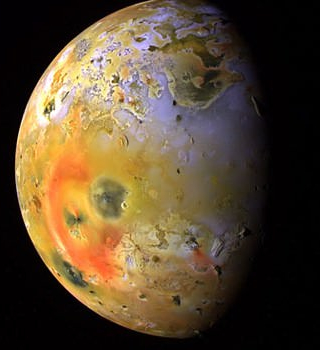
Jupiter’s moon, Io, is by far the most active volcanic body in our solar system. Io raises questions regarding what drives its intense volcanism. Scientists have endeavoured to explain the heat radiated by Io and its interesting surface features. Theoretical models of Io have been developed that imply Io has been recycled by volcanism and tectonics over billions of years. However, it is possible to reinterpret the evidence from Io from a young age perspective.
The age of Io was addressed in my 2003 paper in Proceedings of the Fifth International Conference on Creationism.1 That paper described tidal dissipation and the active volcanism in this moon of Jupiter. It was argued that an age of less than 10,000 years for Io was more plausible than an age of billions of years. Tidal dissipation is a well-known process generating heat in the interior of Io as it orbits Jupiter. The heat is generated from distortion of the shape of the body, more specifically from friction and movement of non-rigid material in the interior. Io radiates approximately 1014 watts of energy in infrared radiation.2,3 In the 2003 paper, I argued that tidal dissipation was not the only heat source in Io and that if Io is understood as only thousands of years old, some of the heat could still be primordial. This paper will update the subject of Io and its volcanism and argue that a young age is still plausible in the light of recent research.
Io is highly influenced by its relative nearness to Jupiter. To compare it to our moon, Io is 6 Jupiter radii from the planet, but our moon is 60 Earth radii from its planet. This, along with the rapid rotation of Jupiter, combines to make the tidal forces on Io very strong. The tidal forces, the orbital motion, and the volcanism on Io are all interacting processes. Io is in a three-body orbital resonance with Europa and Ganymede. This resonance puts the orbital periods of Io, Europa, and Ganymede in a ratio of 1:2:4, where Io is the nearest of the Galilean moons to Jupiter, with Europa and then Ganymede farther out. This resonance causes some periodic variations in Io’s orbit but the tidal effects in Io, Europa, and Ganymede tend to resist the orbital variations and generate internal friction. Scientists have often assumed that Io would migrate outward from Jupiter over time due to the tidal forces, but observations have never really confirmed this.4 Astrometric measurements of Io have not yielded consistent results as to whether Io moves inward toward Jupiter or outward away from Jupiter.
The volcanism in Io is understood as being caused by tidal dissipation heating. Because tidal dissipation is a known, ongoing process, it appears to support the old-age view. In 2003,1 I argued that tidal dissipation is not the only heat source. In 2003, models of Io were falling short by about one order of magnitude from the 1014 W energy output above. I proposed there was primordial heat from creation dissipating in Io in addition to heat from the tidal forces. This paper will re-examine this and other issues about Io.
The age of Io should be considered in relation to the long-term viability of the models of Io’s interior and its volcanism. In my paper,1 a model of the interior of Io was described by Spohn.5 Spohn’s model proposed that large molten magma chambers would build up in Io’s mantle. These magma chambers would drive the volcanism observed on Io’s surface. In models of Io from the early 1990s and prior to 2000, the mantle of Io was assumed to be partially molten, but there was no discussion of liquid layers or ‘magma oceans’ in Io or other moons of the outer solar system. Today, liquid, or partially liquid, layers have been proposed by researchers, to explain Io as well as other moons.6,7 Models of the core of Io were clarified by data from the Galileo mission, which began collecting data about Jupiter and Io from 1995 until the fall of 2003. Gravity measurements from the Galileo spacecraft established that Io does have a metallic core.8 There has long been a consensus among planetary scientists that the core of Io is likely to be a mixture of iron and iron sulfide, which is approximately 850–900 km in diameter (about 50% the total diameter of Io).9 The information we have on Io is data acquired from orbit or from Earth observations. Much of the orbital data comes from the Galileo mission. The Juno spacecraft is currently orbiting Jupiter and is doing new infrared measurements of Io, though Io is not the focus of the Juno mission.
Io volcanism
The Voyager 1 mission photographed erupting volcanic plumes from Io in 1979. The Galileo mission was much better suited than that of Voyager 1 to study the temperature and composition of surface processes related to volcanism. Io’s volcanism is varied in nature. The surface of Io is predominately yellow to red in colour from sulfur materials. The red material is hotter. There is significant white sulfur dioxide as well as elemental sulfur and sulfur chain molecules on the surface. The sulfur changes colour as it cools. However, Io’s overall density is close to that of our moon. Evidence of silicate lavas and ultramafic lavas were found on the surface by Galileo from detection of materials on the surface over 1,000 K in temperature. The hottest molten materials on the surface are the ultramafic lavas (likely containing magnesium), which have been measured to have temperatures reaching 1,870 K.10 The sulfur seems to be driven out as the hotter lavas reach the surface. Liquid lava flows occur on Io as well as some pyroclastic eruptions. The hot basalt and other hot lavas are black unless they are hot enough to glow. Io’s surface appearance was observed to change from comparing the Voyager images to Galileo images and even in comparing images from one Galileo orbit to another. Some volcanic hotspots were discovered from Earth observations after the Galileo mission, and one new hotspot was discovered near the Io South Pole by the Juno spacecraft.11
The black eruption sites on the surface are referred to as paterae on Io. Paterae can be described as caldera-like depressions that have been centres of volcanic eruptions. It has been estimated that about 20% of the paterae observed during the Galileo mission were active during the periods of observation.12 This and photos of the surface suggest there are fault structures that seem to affect where the eruptions take place. Some volcanic sites erupt frequently, while other sites of large eruptions are less frequent. The Loki site (12° N, 310° W) erupts once or twice per year, but it is responsible for about 25% of the heat given off by Io. It is thought to be a large lava lake that covers over, erupting periodically. However, Loki does not have some of the hottest lavas that are present at many other sites. There are also very dramatic eruptions occasionally, usually referred to as ‘outbursts’, that can potentially double the entire heat output of Io for a period of weeks.
The first evidences of volcanism on Io were the volcanic plumes seen by the Voyager spacecraft. Galileo also observed eruption plumes, of differing types. Plume eruptions are always found to be from volcanic sites near Io’s equator. One large volcano on Io with eruption plumes is Pele. The Pele site (22° S, 283° W) plumes contain a mix of sulfur molecules and silicate pyroclastic material. These plumes have reached heights over 300 km above Io’s surface. The heat from Pele is nearly a constant 240 ± 40 GW, yet little lava is seen (figure 1). This suggests the lava is hidden under the surface.3 There are several lava eruption sites on Io that can produce large volumes of lava. The largest eruption of lava in volume observed by the Galileo spacecraft was at the Pillan site, between 7 May and 18 June 1997. Davies, 2001, describes this eruption, comparing it with a notable Earth eruption of 1783 in Iceland:
“In the space of a few months, over 5,300 km2 of Io’s surface was covered with lava to a depth of about 10 m … an eruption volume of 53 km3. The largest terrestrial eruption ever witnessed (in terms of the eruption volume flux) was Laki in 1783 (Thordarson and Self, 1993), which emplaced 15 km3 of lava in a few months.”13
Io research today
Since the Galileo mission to Jupiter, much analysis of Io has been done by researchers. The total heat power radiated by Io (as infrared) is still taken as 1014 W from Io research. Expressed as heat power output per unit surface area, Io’s surface gives off an average of 2.24 W/m2.12,14 This value is roughly 20 times the surface heat flux for the Earth. Today much more is known about the distribution of mountains, hot spots, and lava on Io’s surface. The term ‘hotspot’ for Io refers to the outlet region where a volcanic eruption takes place. This can be related to a volcanic plume or the eruption of molten rock or both.
The Galileo spacecraft measured the magnetic field in the vicinity of Jupiter. Analysis of this magnetic data led to two conclusions: 1) that Io does not have a significant magnetic field of its own, and 2) that there is evidence Jupiter’s magnetic field is inducing a magnetic field in molten material under Io’s surface.16 Scientists modelled the interior of Io in various ways to find what combination of internal layers and mineral properties would fit the Galileo magnetic measurements best. The magnetic field was found to vary in a manner that correlated with Io’s motion, thus implying an induced field in Io. Researchers Khurana et al.15 concluded that the Io interior model which fit observations best treats Io as having an asthenosphere with a global layer of partially molten material at least 50 km thick and which is at least 20% molten. This has been sometimes referred to as Io’s ‘liquid layer’, or as a ‘magma ocean’. But in describing it as liquid this does not mean it is completely molten; it would only be partially molten.
How much can we trust these conclusions regarding the magnetic measurements implying there is a ‘molten layer’ on Io? First, the general magnetic theory behind this measurement technique is sound, and it has been used in analysis of Earth rocks as well as rocks from our moon. At Jupiter, the technique has been used to study all four of the Galilean moons: Io, Europa, Ganymede, and Callisto.16 The Galileo spacecraft made a total of 35 orbits of Jupiter during its mission, but only a few of these orbits were useful for attempting to measure an induced field for Io. In some cases, it flew by Io closer to the poles, which is not conducive to measuring an induced field. In the 2011 paper by Khurana et al., which argues for a global magma ocean in Io’s interior, the authors point out that only four orbits, referred to as I24, I27, I31, and I32, were useful for studying magnetic induction.15 Of these four, detailed plots and discussion is only provided in the paper for the first two of these flybys, I24 and I27. These were cases where the spacecraft passed near to the equator of Io. These researchers compared different models of Io’s interior and plotted the induced magnetic field compared to measurements from the spacecraft. The two most successful models were a hot, solid mantle at 1,400°C and an asthenosphere model with a 20% melt fraction overlying a hot solid mantle. For the I27 orbit case, both models were reasonably good fits to the data. For the I24 orbit case, the asthenosphere with 20% melt was a better fit than the hot, solid mantle model.
An induced magnetic field will show a variation that is periodic with the orbital period and the spin period of the moon. This is the case at Io, but the analysis assumes a global ‘molten’ layer and compares the theoretical induced field to the measurements. Flybys have a weakness in that they may not be able to represent both time and space variations in the field realistically. Good magnetic data of this kind requires many orbits and sensitive instruments in the spacecraft. Thus, it seems questionable to conclude there is a global melt layer based only on one flyby (I24). On the other hand, there must be significant material under Io’s surface conducting an induced current. It seems the data on Io point to a significant amount of molten material under the surface of Io, but whether it is concentrated into one partially molten layer or is distributed in some other way in a solid matrix is not clear.
The picture from researchers emerging today for the interior of Io is that Io has a mantle that is largely solid near the core but contains more melt closer to the top of the mantle. Planetary scientists tend to at least tentatively accept that there is a molten or partly molten asthenoshere layer under the Ionian crust and above the mantle. Estimates of the thickness of the crust range from 11 to 90 km.17 The Io lithosphere is understood to be largely cold and quite solid, except near possible lava ‘pipes’ and faults bringing hot material toward the surface. (Note that the term ‘lithosphere’ refers to something of multiple possible layers, that could include the crust, which reaches the surface.) The lithosphere must be solid because Io does have mountains that are up to 17 km in height. The lithosphere must be able to support the mountains. The molten layer below the lithosphere is thought to be 50 km thick (or more). Magma is thought to rise through the mantle and through the molten layer. Cooling lava tends to be buried by sulfur compounds on the surface. The large amount of lava weighs down the surface and is thought to force material into the melt layer over time. Then, below the melt layer it is assumed there is mantle convection, which brings hot mantle material up to the melt layer. Thus, the melt layer is thought to mix material from the surface and from the mantle. Mantle convection then would carry material from the molten layer down into the mantle again. In this way, it is thought that much of the mass of Io’s mantle, asthenosphere, and crust could be recycled over long periods of time. There is no evidence of a plate-tectonics–type process on Io, thus the above process has been described as a ‘vertical tectonics’.
Io research has directed much attention to studying the surface features. The distribution of volcanic hot spots and the Ionian mountains are significant clues to understanding Io. The Galileo mission also allowed some insight into how Io’s volcanism changes over time. During and after volcanic eruptions on Io, the appearance of the surface can change over periods of a few hours. Some interesting trends have emerged from correlating Earth-based infrared observations with Galileo mission data. First, the distribution of hot spots on Io is usually correlated with the paterae. However, Io’s mountains are not well correlated with the hot spots. Thus, Io mountains are not generally volcanic eruption sites. Sometimes there are fault structures in the vicinity of a mountain where there may be paterae near the mountain, but many hot spots and paterae are not near mountains. Another important fact is that the majority of hot spots and paterae sites are in a belt of latitudes approximately 30° north and south of Io’s equator. However, there are some notable volcanic sites far North of the equator, such as the Chalybes Regio, which is at 55° north latitude.14
Since the volcanism on Io was discovered in the late 1970’s, the physics of tidal dissipation has become better understood. The efficiency of a body such as a moon in generating heat from tidal forces depends on the internal structure and material properties in the body. Our moon is much colder and more rigid than Io, and it is also farther from Earth in comparison to the size of Earth. This makes the tidal dissipation heating much less for our moon. The overall density of Io is not too different from our moon. but Io has a much larger core than our moon and has much more heat energy being transported to the surface than has our moon or Earth, for a given amount of surface area.
Two simple models illustrate how different bodies can respond differently to tidal stresses. Tidal forces create a periodic stress and strain on the minerals of the interior of a solid body. If a body consisted of a liquid iron core surrounded by a solid mantle and lithosphere, tidal dissipation would generate the greatest heating near the poles (of the spin axis). In this first mode, most of the heat would come from solid body tides in the deep interior. On the other hand, if a body had a solid core and mantle with a soft, hot partially molten layer under the crust (as is proposed today for Io), most of the tidal heating manifested at the surface would be in a band surrounding the equator.18,17 In this second mode, the heat comes more from tidal action on fluids in the asthenosphere region. This comes out of computer simulations of the effects of tidal forces. Io seems to blend these two patterns, but with the second heat distribution (heat in an equatorial band) more predominant.
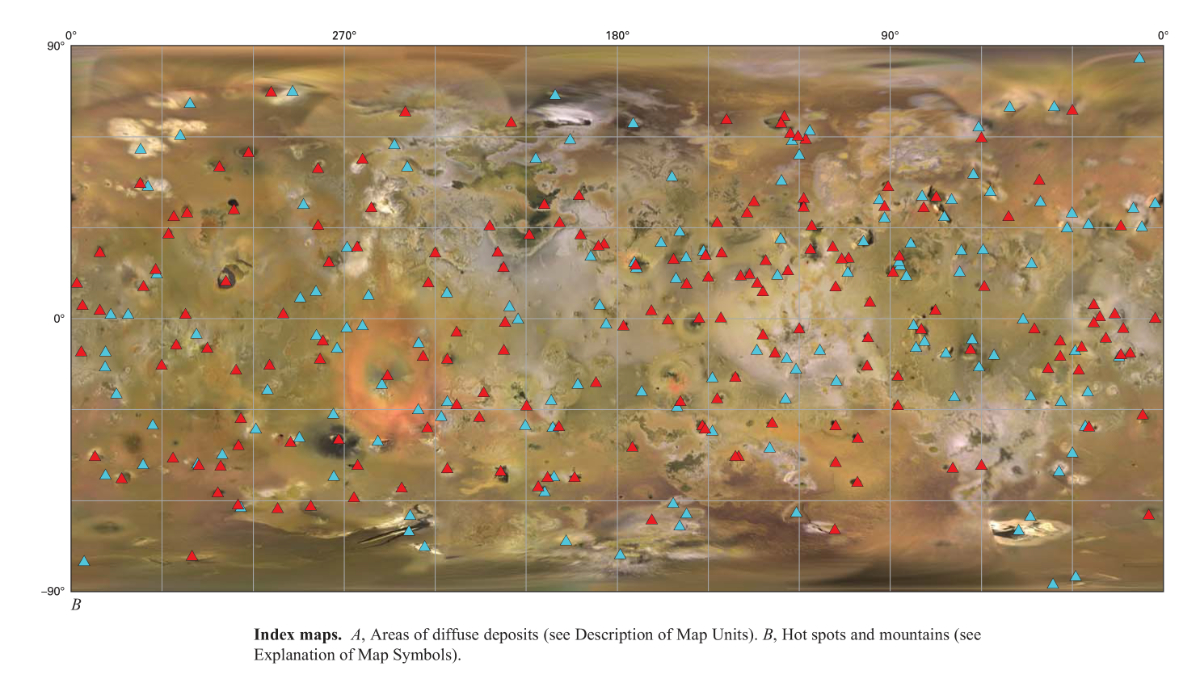
Mountains versus hotspots
The important features of Io’s surface are mountains, patera, and volcanic hotspots. The hotspots are active sources of volcanic eruptions of sulfur materials and/or silicate lavas. The approximate numbers of these features over the entire photographed surface of Io are as follows.12,20
Table 1. Approximate count of mountains, paterae, and hotspots on Io
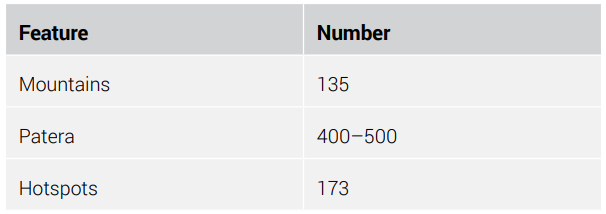
Io patera are similar to volcanic calderas on Earth, but they are not related to mountains in the same manner as on Earth. Alexadra A. Ahern et al. describe paterae this way: “Paterae have steep walls and flat floors that are often covered or partially covered by relatively young lava flow deposits … . The average patera effective diameter (diameter of a circle having the same area as the patera) from a database of 417 paterae is 41 km.”20 As we have seen, ‘hotspots’ refers to active volcanic eruption sites where sulfurous and/or silicate lavas erupt. Many, but not all, of the hotspots are located within the patera depressions. There are many paterae that are not active eruption sites. The fact that the volcanic eruption sites are not located on mountains but are sometimes near them has led to much debate over what the relationship is between the paterae and the mountains. Figure 2 shows a United States Geological Survey map of Io, pointing out mapped hotspots and mountains.21
Io’s mountains are tectonically uplifted structures, often near faults. Io’s mountains are rather different from most mountains on Earth. Ahern et al. provide a useful description of Io’s mountains below. They also point out there is no indication of plate tectonics on Io such as we find on Earth:
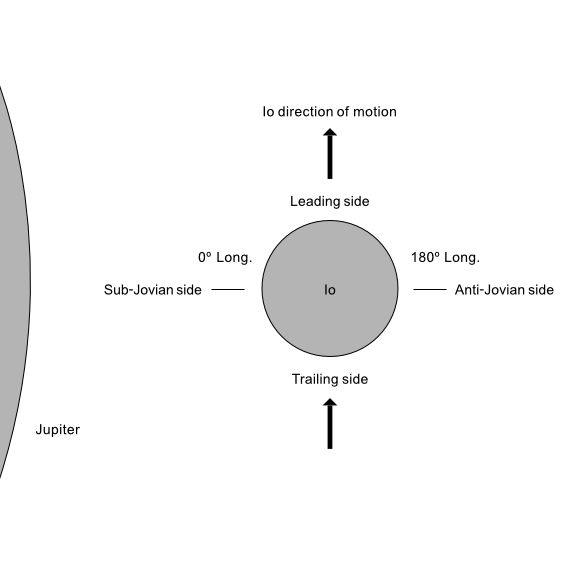
“Despite Io’s persistent volcanic activity, its ~135 reported mountains (Turtle et al., 2001; Turtle et al., 2007) are, with very few exceptions, classified as tectonic rather than volcanic in origin … . They lack distinguishable vents, calderas, or flows emanating from their summits (Turtle et al., 2001) and are blocky and steep-sided, rather than conical. Ionian mountains have been defined by Schenk et al. (2001) as features of positive relief and measurable size higher than 1 km, not solely created by erosional processes. Schenk et al. (2001) measured the average mountain height to be 6.3 km, with Io’s highest peak, Boösaule Montes, rising to 17.5 ± 3 km above the surface … . There was no pattern or alignment of mountains to suggest that a global tectonic regime, such as terrestrial-style plate tectonics, could be responsible for them.”20
There is a tendency for both the mountains and hotspots to be somewhat clustered by longitude on Io. If you were to draw a line through the centre of Io toward the centre of Jupiter, the asthenospheric heating model would predict that the greatest heating around the equator would be where this line meets the Io surface on the side near Jupiter (‘the sub- Jovian point’) and the side opposite Jupiter (‘the anti-Jovian point’). This is in fact the case, except the hottest regions are offset eastward somewhat from this line. Mountains, on the other hand, are somewhat clustered in the opposing manner, near the leading and trailing sides of Io, toward and away from its direction of motion in its orbit (see figure 3). Though the relationship between the paterae and the mountains is not well understood, it is clear tidal forces in Io have had a significant effect on the surface structures and the hotspots.
Io’s surface is definitely young. No impact craters are visible anywhere on the surface, likely because of the resurfacing. The patera structures themselves would probably have a limited lifetime as well because new eruptions could melt the patera walls, cause them to collapse, or cover them, over time. It has been estimated many structures on the surface of Io would only have a ‘lifetime’ of approximately 1 million years.13,10,20 Also, Laszlo Keszthelyi et al. estimate the time necessary to form the paterae structures, “Since most paterae are hundreds of meters to a few kilometers deep, this suggests that patera formation typically takes on the order of 102–105 years.”10
Heat distribution
Scientists have considered the problem of what mechanism could transfer adequate heat to the surface. To address this, a concept was proposed in 1981 that scientists seem to still follow variations on today.22 This proposed there were volcanic vents or dikes that transported lava vertically through a thick Io lithosphere, and below the lithosphere was a partially molten mantle. This is much like today’s approach, but the Galileo magnetic measurements suggested a melt layer below the lithosphere containing more melt than the mantle below it. The large volumes of lava that spread out over the surface are understood to force material down into the mantle over time. The exact nature of Io’s mantle is still debated.
Of Io’s 173 active hotspots that have been mapped, above, 92 lie in the band of latitudes from 30° south to 30° north. These are the most frequent and persistent eruption sites. Those north of 30° N latitude were 38 and those south of 30 S latitude were 43. Thus, there are a significant number of hotspots that are not in the equatorial band of latitudes. In addition, Io has occasional large volcanic events that are short-lived (the outbursts).
Cantrall et al., 2018, summarized geologic associations of surface features and volcanic activity from 2001 to 2016.14 This study brought together data from both Earth telescope infrared observations and the Galileo mission. Cantrall et al. state, “Volcanic outbursts on Io represent the most powerful eruptions in the Solar System.”14 This same study indicated that over both the Galileo mission years and the 15 years of Earth observations, Io’s global heat flux was largely constant, overall. These researchers comment on the source of the magma driving Io’s volcanism:
“While our observations show the greatest heat flux from low to mid-latitudes, they also suggest a significant magma source feeding higher latitude regions. … Together, these observations indicate that heat dissipation is also occurring at depths beyond the asthenosphere and more likely in the mantle.”14
A.G. Davies et al. analyzed Galileo mission results and found that the heat from Io’s hot spot volcanic centres accounts for about 54% of the total output of Io as determined from Earth ground-based thermal infrared measurements.3 This raises the obvious question concerning the other 46%. Davies et al. argue it is coming from the Io poles. They point out evidence for this from the Galileo photopolarimeter radiometer (PPR). This instrument looks at the degree of polarization of reflected light and measured the intensity of infrared radiation from Io:
“Galileo PPR data revealed that Io has anomalously warm surfaces at high latitudes (Rathbun et al., 2004), showing that nighttime brightness temperatures at latitudes between 60° S and 75° S ranged from ~95 K to ~90 K. This temperature is higher than the expected 80 K to less than 70 K.”3
A.G. Davies et al. argue that if the 90 to 95 K temperatures covered the northern and southern portions of the surface from about 43° or 48° north latitude up (and similarly in the south) then this could account for the 46% of Io’s heat output.3 They go on to say, “If all of the unaccounted for energy is being lost from Io’s poles, then this would imply a ratio of shallow (aesthenospheric) to deep (mantle) heating of about 1:1.”3 This shows the heat output on Io’s surface is not only following the asthenospheric models with heat from the equatorial band, but heat is also coming from the polar regions. In Io, the lower mantle seems to be more rigid and has less molten material than the upper mantle. This would make the tidal dissipation mechanism less efficient in the lower mantle in generating heat, based on theoretical models. Thus, scientists have found it somewhat surprising that Io has hotspots nearer to the poles. The deep mantle heat may also be from tidal dissipation, or some of it may be heat from creation. The upper mantle heat source has likely developed in its current configuration due to ongoing tidal forces and volcanism.
In Spencer, 2003, it was argued that observations of infrared emissions from Io were about one order of magnitude larger than what could be explained from tidal dissipation heating. However, the issue of whether the models have been able to match the observed radiated heat output of Io is still debated among researchers today. In Valery Lainey et al., from 2009, it was argued that their tidal model for the heat output matched well with the observations from the orbit astrometric measurements.23 This study is the most recent astrometric orbit analysis of Io. However, the methods in this study are out of date. First, they did not use Galileo mission data due to a problem in Galileo’s high-gain antenna. They also neglected the tidal dissipation in Europa and Ganymede. Also, some of the assumptions in their analysis are considered inaccurate today, such as that the tidal dissipation Quality Factor (Q) of Jupiter is a constant.
In an important research report from 2019 (of which Valery Lainey was a coauthor), Katherine de Kleer et al. comment on the Lainey 2019 results.24 They point out that if the tidal dissipation of Europa and Ganymede are included, the tidal heat figure becomes 0.5 x 1014 W, which is less than the observed value. More recent studies (such as Beuthe18 and Tyler19) attempt to better account for the internal properties of the body. Tyler et al. indicated they could match the surface heat flux of 2.25 W/m2 under certain conditions.18 Today’s tidal models fit observations better than those of 2003, but there is still debate as to whether theoretical tidal dissipation calculations can adequately match the observed heat output.3 Yet for considering the age of Io, there may be a more important issue. Are today’s models of Io plausible over billions of years?
Io over billions of years
In today’s models of Io, the moon must recycle itself. This means that Io must have been completely resurfaced many times in the history of the solar system, including a process of destroying and rebuilding the mountains. Keszthelyi et al. aptly summarize the magnitude of the problem. These comments were made not long after the end of the Galileo mission:
“If Io has been as volcanically active as it is today (erupting ~550 km3/yr. of lava (Blaney et al., 1995) for the past 4 billion years, it has produced a volume of lava equivalent to ~140 times the volume of Io. … The observed enstatite-rich, high-melting-temperature lavas should not exist on a highly differentiated Io. The only way such lavas could exist is to efficiently recycle the crust into the mantle.”10
This figure of 140 times the volume of Io presents a problem for assuming Io is very old. In recycling a moon, likely the core would not participate since it is not feasible to ‘remix’ the core with mantle material. Galileo gravity measurements estimated that Io’s core accounted for about 14% of the mass of Io.9 Thus, leaving the core mass out of the recycling could increase the 140 figure above to 160 times the portion of Io participating in the recycling. Also, the quote above comments on high-melting-temperature lavas. One would expect that after many cycles of remixing into the mantle and volcanism driving material back to the surface, the higher-density minerals would stay in the lower mantle and the composition of the lavas reaching the surface should become more uniform over time. Yet the spectra and temperatures observed on the surface imply the lavas are of varied compositions.
This recycling of Io’s entire mass 140 times in over 4 billion years appears to be taken on faith by scientists today. The possibility of Io being only several thousand years old is not considered in the above comments. Yet, assuming a young age simplifies explaining Io. On evaluation, recycling the entire surface of Io would require a process to destroy and rebuild the mountains many times! There is no seismic data from Io to verify any of the subsurface structure that models have proposed. The volume of lavas on Io is also very nonuniform, being much greater in certain sites in the equatorial regions than in the northern or southern latitudes, most of the time. This raises the question as to whether a partially molten layer would be stable over long periods of time. Some form of dikes or lava vents bringing the large volumes of lava to the surface is plausible and is somewhat similar to evidence for volcanism on our moon. But currently there is no way to verify if material from the crust is mixing back into the mantle.
A young-age view of Io eliminates the need to explain how a mass equivalent to most of the mass of Io could be recycled many times. In a young-age view, the volcanism could be essentially one way. There is some evidence suggesting large magma chambers under Io’s surface (such as Loki). Presumably, these do not remix into the mantle because they are not dense enough to sink. There is not a clear explanation of how the remixing into the mantle would take place. Only the assumption that the weight of the lavas would accomplish it.
Conclusions
Though research on Io has progressed since 2003, a young-age view is still plausible and indeed less problematic. Today, I would consider a global partially molten layer under the lithosphere to be plausible, but not necessarily the only possible configuration for the molten material. But the distribution of molten material under the surface of Io is not critical to the question of the age of Io. The recycling of material through volcanism and mixing with the mantle is more the real problem. Io does have lithospheric blocks or what one could call ‘plates’ (which support the mountains) but there is no evidence of plate tectonics. Though Io’s most active volcanic sites are generally within a band near the Io equator, there are also volcanic sites at high latitudes. Tidal dissipation heating appears to be at work both in the deep mantle of Io and in the upper mantle.
Today, scientists have been able to construct theoretical interior models that produce at least close to the observed heat output. But even with this apparent success, the implications of the models require a process that would recycle the volume of Io 140 times in over 4.5 billion years. All the mountains on Io would have to have been broken up and recycled into the mantle, then rebuilt repeatedly. There is evidence of some uplift of mountains along fault structures. The large volume of lavas likely creates stresses that cause some isostatic readjustment of the surface. But to infer from this that the entire moon has been recycled over and over is unreasonable. It is clear that the surface of Io is relatively young. Thus, the entire moon can be interpreted as young without contradicting known facts. Io is unique among moons in our solar system. It provides a useful laboratory for the study of tidal effects and orbit resonance. Io was apparently created to be a volcanic powerhouse which demonstrates God’s creativity.
References and notes
- Spencer, W., Tidal dissipation and the age of Io; in: Ivey, R.L. Jr (Ed.), Proceedings of the Fifth International Conference on Creationism, Creation Science Fellowship, Pittsburgh, PA, pp 585–595, 2003. Return to text.
- Veeder, G.J., Matson, D.L., Johnson, T.V., Blaney, D.L., and Goguen, J.D., Io’s heat flow from infrared radiometry: 1983–1993, J. Geophysical Research 99( E8):17095–17162, 25 1994. Return to text.
- Davies, A.G., Veeder, G.J., Matson, D.L., and Johnson, T.V., Map of Io’s volcanic heat flow, Icarus 262: 67–78, 2015. Return to text.
- Lieske, J.H., Galilean satellite evolution: observational evidence for secular changes in mean motions, Astronomy and Astrophysics 176:146–158, 1987. Return to text.
- Fischer, H.J., and Spohn, T., Thermal-orbital histories of viscoelastic models of Io (J1), Icarus 83:39–65, 1990. Return to text.
- Kivelson, M.G., Khurana, K.K., Russell, C.T., Volwerk, M., Walker, R.J., and Zimmer, C., Galileo magnetometer measurements: a stronger case for a subsurface ocean at Europa, Science 289(5483):1340–1343, 2000. Return to text.
- Spencer, W., Warm icy moons, J. Creation 29(3):53–59, 2015. Return to text.
- Anderson, J.D., Sjogren, W.L., and Schubert, G., Galileo gravity results and the internal structure of Io, Science 272:709–712, 1996. Return to text.
- Kuskov, O.L. and Kronrod, V.A., Core sizes and internal structure of Earth’s and Jupiter’s satellites, Icarus 151:204–227, 2001. Return to text.
- Keszthelyi, L., Jaeger, W.L., Turtle, E.P., Milazzo, M., and Radebaugh J., A post-Galileo view of Io’s interior, Icarus 169:271–286, 2004. Return to text.
- Mura, A., Adriani, A., Tosi, F. et al., Infrared observations of Io from Juno, Icarus 341, paper 113607, in press, 2020. Return to text.
- Hamilton, C.W., Beggan, C.D., Still, S. et al., Spatial distribution of volcanoes on Io: implications for tidal heating and magma ascent, Earth and Planetary Science Letters 361:272–286, 2013. Return to text.
- Davies, A.G., Volcanism on Io: the view from Galileo, Astronomy and Geophysics 42(2):2.1–2.15, 2001. Return to text.
- Cantrall, C., de Kleer, K., de Pater, I. et al., Variability and geologic associations of volcanic activity on Io in 2001–2016, Icarus 312:267–294, 2018. Return to text.
- Khurana, K.K., Xianzhe, J., Kivelson, M. et al., Evidence of a global magma ocean in Io’s interior, Science 332:1186–1189, 2011. Return to text.
- Saur, J., Neubauer, F.M., and Glassmeier K.H., Induced magnetic fields in solar system bodies, Space Science Reviews 152(1–4):391–421, 2010. Return to text.
- Knicely, J., Everett, M.E., and Sparks, D.W., Magnetic anomalies on Io and their relationship to the spatial distribution of volcanic centers, Physics of the Earth and Planetary Interiors 269:133–147, 2017. Return to text.
- Beuthe, M., Spatial patterns of tidal heating, Icarus 223:308–329, 2013. Return to text.
- Tyler, R.H., Henning, W.G., and Hamilton, C.W., Tidal heating in a magma ocean within Jupiter’s moon Io, The Astrophysical J. Supplement Series 218:22–38, 2015. Return to text.
- Ahern, A.A., Radebaugh, J., Christiansen, E.H. et al., Lineations and structural mapping of Io’s paterae and mountains: implications for internal stresses, Icarus 297:14–32, 2017. Return to text.
- Williams, D.A., Keszthelyi, L.P., Crown, D.A. et al., Geologic map of Io, Scientific Investigations Map 3168, U.S. Geologic Survey, 2011. Return to text.
- O’Reilly, T.C. and Davies, G.F., Magma transport of heat on Io: a mechanism allowing a thick lithosphere, Geophysical Research Letters 8:313–316, 1981. Return to text.
- Lainey, V., Arlot, J.-E., Karatekin, O., and Hoolst, T.V., Nature 459(7249):957–959, 2009. Return to text.
- de Kleer, K., McEwen, A.S., Park, R.S. et al., Tidal heating: lessons from Io and the Jovian system—final report, Keck Institute for Space Studies, June 2019, researchgate.net/publication/336751131. Return to text.
Readers’ comments
Comments are automatically closed 14 days after publication.