Journal of Creation 31(2):119–127, August 2017
Browse our latest digital issue Subscribe
Non-glacial landforms indicate thin Scandinavian and British-Irish Ice Sheets
Just like the Laurentide Ice Sheet, the Scandinavian and British-Irish Ice Sheets were much thinner than the Antarctic Ice Sheet, which is used as an analogue for ice sheet thickness by uniformitarian scientists. This supports a higher sea level minimum in the creation science Ice Age model. The evidence for much thinner ice sheets in Europe includes tors, relatively thick soils, saprolite, blockfields, and slightly modified drainage features within the area covered by the ice sheets. These non-glacial landforms are even found at the proposed centre of the Scandinavian Ice Sheet over north-east Sweden that extends into northern Finland. To preserve such landforms, the ice sheet in these areas must have eroded the substrate very little over a few million years. Secular scientists are convinced the Scandinavian Ice Sheet was up to 3–4 km thick, and are forced to conclude these areas had been covered by cold-based ice, which causes little erosion. Nunataks are supposed to have protruded above the ice sheets, but uniformitarian scientists are forced to claim that the trimlines on nunataks are the boundary between warm ice below and cold ice above, instead of the boundary between ice below and no ice above. Some of the delicate pre-glacial features can potentially be explained by late Flood events and the unique post-Flood Ice Age.
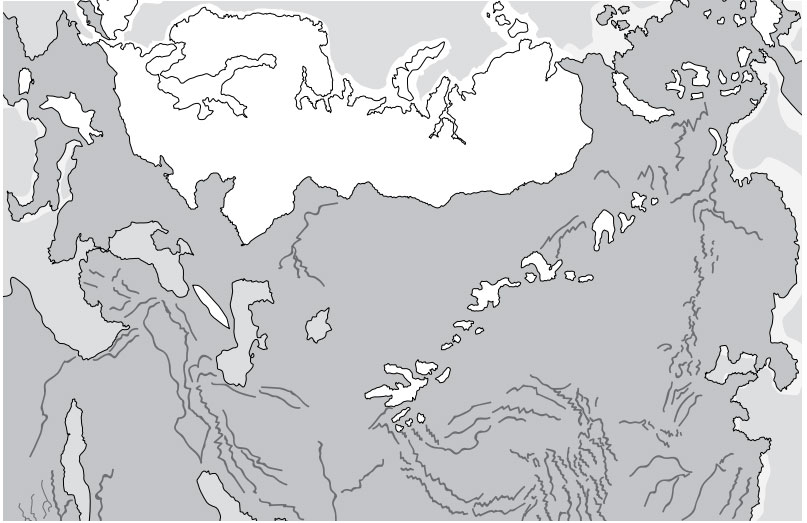
Uniformitarian scientists commonly believe ice sheets during the Ice Age were the same thickness as the existing Antarctic Ice Sheet.1,2 These former ice sheets include the Laurentide Ice Sheet over central and eastern Canada and the adjacent United States, the Cordilleran Ice Sheet over western Canada and the adjacent United States, the British-Irish Ice Sheet over the United Kingdom, and the Scandinavian (Fennoscandian) Ice Sheet over northern continental Europe and north-west Asia. However, a great deal of evidence now suggests that the Laurentide Ice Sheet, the largest, was much thinner than the ice sheet in Antarctica.3 In this paper, further evidence will be presented that the Scandinavian and British-Irish Ice Sheets were also much thinner than Antarctica.
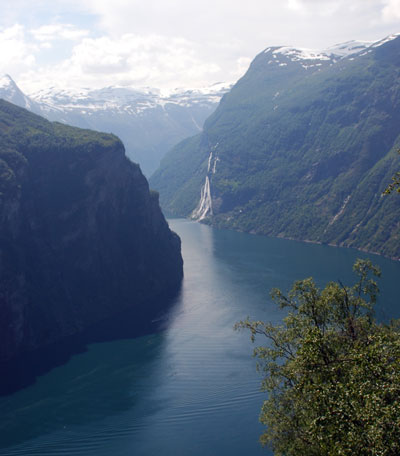
The Scandinavian Ice Sheet
The Scandinavian Ice sheet once covered northern continental Europe and extended into north-west Asia, including north-west Siberia (figure 1). The large islands north and north-east of Scandinavia, like Svalbard,4 were also glaciated. Researchers now believe the shallow Barents Sea and other seas were partly-to-totally glaciated as well.5–8
Secular scientists claim there were 40–50 ice ages separated by interglacials in the Pleistocene9 because of their acceptance of the astronomical or Milankovitch theory of ice ages. (The claim of 40–50 ice ages comes mainly from oxygen isotope variations in deep-sea cores.) Secular scientists argue that, during each ice age, snow and ice began building up over the Scandinavian Mountains. This they also argue started about 2.5–2.8 Ma,9 but it was not until about 1 Ma or later that some scientists think the snow and ice centre for each subsequent ice age spread out of the mountains into north-eastern Sweden, becoming 3–4 km thick.10 They also believe an ice divide extended eastward from north-east Sweden over the high terrain of northern Finland.11
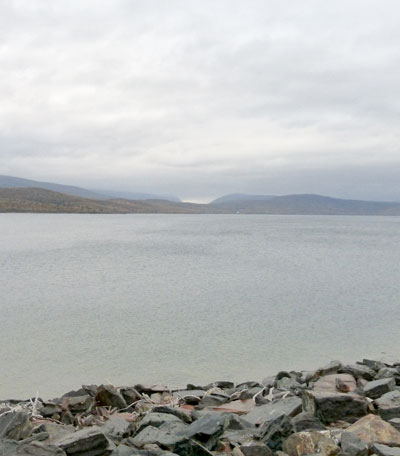
The last glaciation is thought to be one of the largest. Since each ice age apparently reworks and destroys much of the evidence of previous ice ages,10 we mostly only have terrestrial evidence for the last ice age. This argument, of course, does allow a scientist to add as many ice ages as desired or required. The last glacial maximum (LGM) is said to be about 20,000 years ago, with deglaciation thereafter to about 10,000 years ago.12 These dates are based on the Swedish varve chronology,10 which is a problematic chronology.13,14
The Ice Age or ages created fjords15 along the west coast of Norway (figure 2). On the eastern slopes of the Scandinavian Mountains are valleys and foothills called the piedmont. The valleys commonly contain deep, long lakes (figure 3). The piedmont lakes are almost as deep as the fjords and were carved the same way.10 Major end moraines lie just east of the mountains, while lateral moraines are common in the piedmont valleys. The estimated thickness and volume of the Scandinavian Ice Sheet at the LGM varies widely among researchers.16
Ice scouring is easily deduced where the Scandinavian Ice Sheet once was, as indicated by drumlins and other linear streamlined hills. These features are not correlated with bedrock lithology,17 indicating that they were carved by ice. These scoured features are now considered parts of ice streams (a glacier that moves more than 1 km/yr18) commonly inferred for the ice sheets during the Ice Age.19
British-Irish Ice Sheet
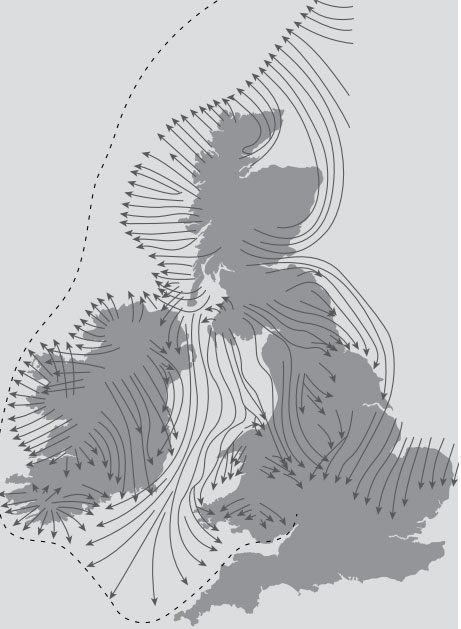
The British-Irish Ice Sheet (figure 4) is now considered mostly separate from the Scandinavian Ice Sheet, divided by the Norwegian Channel off the west coast of Norway. It is thought to have once been connected to the Scandinavian Ice Sheet but disconnected during deglaciation.20,21 Until recently, the ice sheet was thought to have been much less extensive than shown in figure 4, but it is now argued that the British-Irish Ice Sheet covered all but southern England. Although the southern configuration of the ice sheet on Ireland is unknown and controversial,22 it is now believed that all of Ireland was glaciated, with the presence of a secondary local ice cap in the south-west mountains (figure 4). There was also a separate ice cap on the Outer Hebrides and the Shetland Islands 20,23,24 that merged with the ice moving west off north-west Scotland. This ice sheet is thought to have been two-thirds marine in the shallow seas with large ice streams. This was deduced from detailed features on land and on the ocean bottom.24,25
Non-glacial landforms
A variety of non-glacial landforms, which formed either before glaciation or during an ‘interglacial’, and which did not form after glaciation, are found in previous Pleistocene glaciated areas. This suggests that little erosion occurred with the supposed succession of ice sheets. These landforms also cast doubt on much of the uniformitarian ice age paradigm, especially its long timescale of up to 2.6 Ma associated with the general start of the Pleistocene glacial/interglacial oscillations. These non-glacial landforms observed in the area covered by the Scandinavian and British-Irish Ice Sheets include tors, relatively thick soils, saprolite, blockfields, and little modification of pre-glacial drainage features.16 Nunataks also protruded above the ice until recently (discussed later).
Tors
A tor is “A high, isolated crag, pinnacle or rocky peak; or a pile of rocks much-jointed and usually granitic, exposed to intense weathering, and often assuming peculiar or fantastic shapes.” 26 Figure 5 shows a tor from non-glaciated Dartmoor, south-west UK. Tors are especially significant because they can be easily eroded by a glacier or ice sheet, and therefore are an indication that there was little erosion in the area. However, this conclusion could be somewhat modified if the tor was altered by erosion.27 Hättestrand and Stroeven declare: “The implication is that the tors predate the oldest recorded ice flow in the area because they are not resistant to glacial erosion.”28 Tors are mostly considered pre-glacial or non-glacial landforms.29
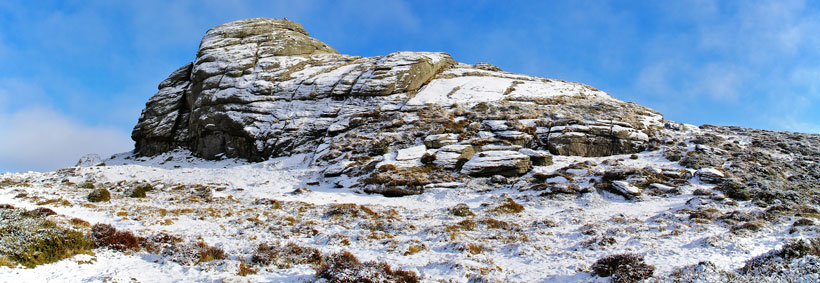
Saprolite
A saprolite is “A soft, earthy, typically clay-rich, thoroughly decomposed rock, formed in place by chemical weathering.”30 It is generally interpreted as weathered bedrock. Thick soils and saprolites are considered pre-glacial weathering features,25,27 since weathering and soil development in previously glaciated areas has been limited.31 Glaciers and ice sheets should easily erode soils and saprolites since modern glaciers are very erosive.32
Blockfields
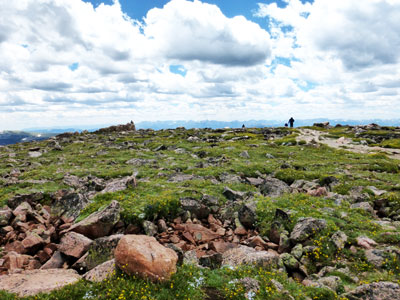
A blockfield is “A thin accumulation of usually angular blocks, with no fine sizes in the upper part, over solid or weathered bedrock, colluvium, or alluvium, without a cliff or ledge above as an apparent source.”33 Blockfields are commonly found on low-relief mountaintops (figure 6) or plateaus.
The origin of blockfields is somewhat of a mystery, especially since they are neither forming today nor did they form after the ice left, with the exception of limestone blocks in the Canadian Arctic.27,34,35 Many researchers thought that blockfields formed before glaciation in a warm pre- Pleistocene climate.36,37 However, the consensus has shifted to viewing them as a result of a cold environment associated with glaciation.35,38–41 Because occasional erratic boulders and meltwater channels are associated with blockfields, and there is no other evidence of glaciation, researchers now think ice actually covered the tops of the mountains but was cold-based and did not erode the blockfield. Cosmogenic isotopes indicate the blockfields survived at least the last ice age. Regardless, Goodfellow et al. think they may never have been eroded by glaciers: “Autochthonous blockfield mantles may indicate alpine surfaces that have not been glacially eroded.”42
Nunataks
A nunatak is “An isolated hill, knob, ridge, or peak of bedrock that projects prominently above the surface of a glacier and is completely surrounded by glacier ice.”43 The line on the nunatak, which shows erosion below and none above, is called the trimline. The existence of such features raises many questions with regard to both traditionally argued and the more recently argued cold ice sheets.
Survival of delicate landforms
Non-glacial landforms left by the Scandinavian and British-Irish Ice Sheets are found in abundance. These should have been easily eroded by glaciation.44 Therefore, it is difficult for uniformitarian scientists to explain them with their proposed 40–50 ice ages.
Scandinavian Ice Sheet
Non-glacial features extensively cover the area of what was once the Scandinavian Ice Sheet, including where ice was supposed to be thick over northern Sweden and Finland.19,45,46 For instance, tors are common in arctic Finland.47 Tors and saprolites are common in areas of north-east Sweden, indicating glaciation had little effect.48,49 However, they are also adjacent to areas of significant glacial erosion, as shown by streamlined landforms suggestive of ice streams.19,25,49 In areas where tors and saprolites occur, pre-glacial drainage was hardly modified, as would be expected with so many glacial/interglacial cycles over 2.5 to 2.8 Ma. Even in glacially streamlined areas, planation surfaces and inselbergs that were only slightly planed, or not planed at all, still exist. This shows that erosion by the ice streams was insignificant. Although there are patches of thick till in northern and central Sweden,9 glacial till is generally thin.31 This is anomalous considering all the proposed glacial activity. The glacial transport of eroded rocks was also of short distances.50 Indeed, Stroeven et al. exclaim:
“For a site near the centre of Fennoscandian glaciation, it is remarkable that the landscape is characterized by well-developed tors, boulder fields, and weathering mantles, and that the only traces of glaciation are till veneers and meltwater channels.” 51
This is truly remarkable, and points more to a problem with the paradigm:
“Preservation of extensive and deep Neogene [late Cenozoic] weathering requires that cold-based patches develop in the same areas repeatedly and throughout the many cold stages of the late Pleistocene (Hall, 1986).”52
When such contrived conditions are required to prop up the ‘multiple ice ages’ idea, it is no surprise that researchers admit that they do not understand glacial erosion and deposition in Scandinavia.9
Twenty percent of the mountains of northern Sweden that were repeatedly glaciated have non-glacial or pre-glacial landforms, including tors.32 Even heavily scoured areas show minimal erosion. Glacial erosion seems to be mainly linear, enlarging pre-glacial valleys into fjords and piedmont troughs in western Scandinavia.
Although some earlier researchers claimed deep erosion by ice sheets,53,54 many other researchers say that little glacial erosion has taken place.19,55 They point to saprolites with little glacial weathering that occur in central Sweden.55 Planation surfaces with inselbergs are found not only in central Sweden but also over much of Scandinavia (figure 7) at various altitudes.19,56 They indicate little glacial erosion.57,58 The mountains of Norway also show little glacial erosion at the tops, while the main glacial erosion is linear in fjords.59 Most landforms are pre-glacial.60 The east slopes of the mountains of western Scandinavia also show little erosion.9
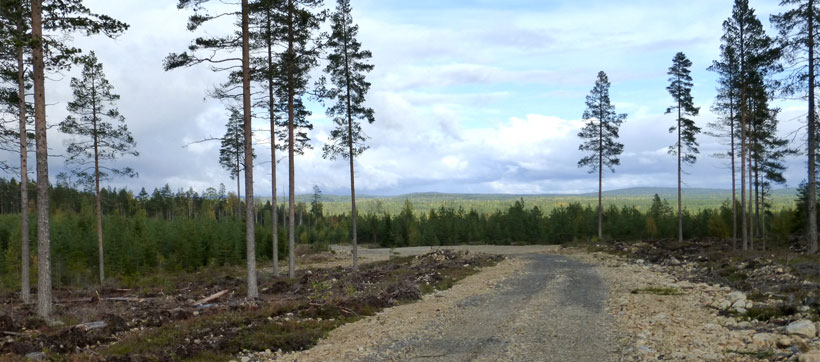
The British-Irish Ice Sheet
There is little glacial erosion in the North-West Highlands of northern Scotland (figure 8), as shown by tors and other non-glacial features.44,61,62 For instance, tors and blockfields are found in the Caithness Mountains of north-east Scotland.63 Saprolites up to 60 m (200 ft) thick that are thought to represent a warm, humid climate survive in north-east Scotland.64,65 Blockfields and nunataks in the mountains and plateaus of north-west Scotland show little glaciation, although rare exotic boulders transported by ice occur on some mountains,62,66 which have caused researchers to suggest these high areas were covered by ‘cold-based’ ice.
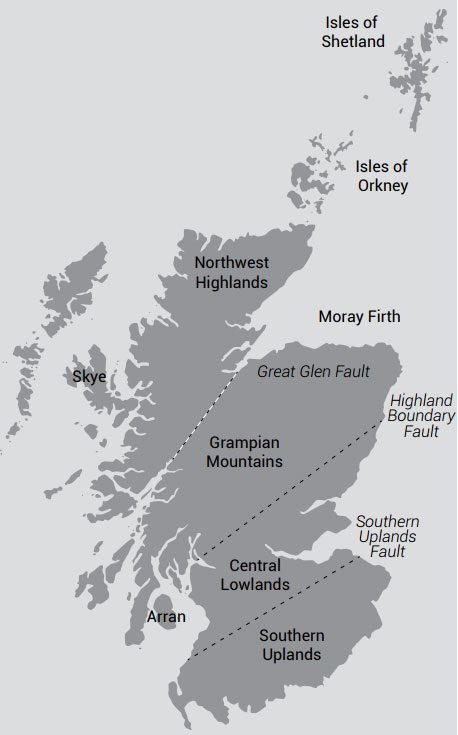
Farther south, tors are common in the Grampian Mountains of central Scotland.44 These mountains cover about half of Scotland. There are a lot of impressive tors up to 15 m (50 ft) high on the Cairngorms Mountains, part of the eastern Grampian Mountains. Some tors have been slightly modified by glacial flow.67 Tors are found at mountaintops of Arran Island of west-central Scotland, as well as elsewhere in Scotland.68
In the Southern Uplands of southern Scotland (figure 8), there is little evidence of glaciation in the Cheviot Mountains of south-east Scotland and adjacent England, as shown by areas of deep weathering and tors.69 Evidence of intense erosion does occur at lower elevations around the Cheviot Mountains that are attributed to ice streams.
Ice moved radially from an ice cap over the Lake District of north-west England, which was thin with nunataks and blockfields protruding above the ice.70 Farther south, deeply weathered material and several planation surfaces exist in Wales in areas that were glaciated.71 Tors are recorded in south-west Wales.63 Ollier states that many pre-glacial Tertiary features are preserved in the British Isles.72 There is little evidence of glaciation on the higher plateaus of Ireland, as shown by blockfields.66 Deep weathering occurs in north-east Ireland that was not erased by glaciation.73
Rescuing hypotheses unlikely
If scientists objectively investigated all this evidence, they would naturally conclude that the Scandinavian and British-Irish Ice Sheets were thin and that there was only one ice age. However, secular glaciologists assume there have been 50 ice ages of various intensities in regular succession beginning about 2.6 Ma74 because they assume the astronomical theory of ice ages. In the Scandinavian Ice Sheet, the thickest ice sheets are believed to have built up to about 4 km over northern Sweden and Finland, although some researchers admit that they do not know its thickness and volume.75 However, the astronomical theory is fraught with problems.76–79 The main difficulty is that the mechanism is weak, so researchers are constantly looking for a secondary mechanism to boost its strength.10
Cold-based ice sheets
Because of their uniformitarian assumptions, secular scientists are forced to assume that each ice age was ‘cold-based’ at the same locations where the non-glacial features are located.9,25,51 This is because the lower levels of a cold-based ice sheet do not move and are frozen to the bed. Without ice motion, delicate features such as non-glacial features would not be eroded. For instance, tors are claimed to have survived many such glacial cycles.80
But how likely is it that ‘cold-based’ formation took place in the same areas up to 50 times? Although it is not expected that all these ice ages necessarily affected the same area (some were smaller and some were larger), even the smaller ice ages would have at least glaciated the mountains of Scandinavia. It is improbable that each ice age would be cold-based at the same location, especially since many uneroded areas are adjacent to areas scoured by ice streams.49 Did ice streams always develop at the same locations? Although some ice streams are guided by bottom topography, some are random.81 These random ice streams would be expected to erode all non-glacial landforms if there were 50 ice ages. Ebert expresses great surprise:
“Scandinavia is unique in the respect that pre-Quaternary landforms were repeatedly covered by Quaternary ice sheets but often survived with different degrees of glacial modification.” 82
But even if there was just one ice age, it should erode these non-glacial landforms. As an ice sheet first develops and then melts back, it would move since it would have a surface slope (one of the variables in glacial movement). Cold-based areas should be rare early and late in an ice age, when the ice is relatively thin and summers relatively warm. And even cold-based ice can still move and be erosive.27 Thus, delicate pre-glacial features should be erased with just one thick uniformitarian ice sheet.
Nunataks did not exist
Because secular scientists believe in thick ice sheets, they believe nunataks really did not protrude above the ice, but instead were covered by non-erosive, cold-based ice above the trimline.38 Glaciologists had universally inferred trimlines to be indicators of maximum ice thickness— until recently. Models of glaciation resulting in thick ice sheets have many uniformitarian assumptions and indicate nunataks must have been glaciated.40 However, cold-based ice forming on the tops of the mountains in Ireland, Scotland, England, and Scandinavia is implausible because the 40 or more ice ages most likely started in the mountains adjacent to the relatively warm North Atlantic Ocean. So surely the delicate mountaintop blockfields would have been eroded during mountain ice buildup.
The blockfields with erratic boulders and meltwater channels probably represent the height of the ice in the adjacent valley. Erratics and channels with no other signs of glaciation could form on low-relief mountaintops when the top of the ice sheet melted. Nunataks with tors and blockfields still point to a thin ice cover in contrast to uniformitarian estimates.
The ice sheets had much less ice
All the evidence for the preservation of non-glacial features indicates that the Scandinavian and British-Irish Ice Sheets were much thinner than uniformitarian scientists believe. The ice was not thick enough to erode all the delicate surficial features. Ice was either non-existent (true nunataks) or shallow on the tops of the mountains, and confined mainly to the valleys. The ice centre was not over northern Sweden and Finland but over the western Scandinavian Mountains, as deduced from the terminal and lateral moraines in the piedmont valleys. The ice likely thinned considerably eastward into Sweden and Finland. The Scandinavian Ice Sheet was likely composed of multiple, thin domes, causing it to spread from these domes in ice streams, leaving behind uneroded non-glacial landforms between ice streams. Erosion was minimal even in areas scoured by ice streams. So, the volume of the Scandinavian Ice Sheet must have been much less than that suggested by secular scientists.
The evidence indicates that the main erosion was linear along the western edge of the ice sheet close to the warm North Atlantic Ocean and in valleys east of the mountains. The Scandinavian Mountains are where heavy snow would be expected. It is also where fjords or over-deepened valleys are located. Fjords likely represent a glacially over-deepened pre-glacial valley and are now inundated by the sea. (The over-deepening was likely caused by intense basal meltwater erosion since water pressure is proportional to ice thickness. This would explain why fjords are deepest close to the mountains and shallow seaward.)
The evidence for thin ice over eastern Sweden and Finland also conflicts with the deduction of thick ice based on isostatic uplift, as deduced by high shorelines and geodetic measurements showing an uplift of nearly 1 cm/ yr in the Gulf of Bothnia. Isostasy is beyond the scope of this article, but still needs to be explained in light of the geomorphological evidence considered in this paper.
Support for the biblical Ice Age
The delicate pre-glacial features support a rapid post- Flood Ice Age caused by Noah’s Flood.83,84 This Ice Age would have been roughly 700 years long with thinner ice sheets than what secular scientists believe. Some of the non-glacial landforms, such as valleys and pre-glacial drainage features, can be explained by erosion during the Recessive Stage of the Flood 85,86 and post-Flood weathering during a unique Ice Age. Tors would simply have formed somewhat analogously to inselbergs (an isolated hill rising from a plain) left from Flood runoff, but of much smaller height.
Other delicate features are more difficult to explain in the biblical framework, but do have potential solutions. For instance, soils can be either mud left over from the drainage of the Floodwater and/or weathering early in the Ice Age, especially at lower elevations. Post-Flood weathering would be rapid in the warm, humid climate early in the Ice Age—a result of warm oceans at mid and high latitudes. The warm onshore flow of moist air would especially bathe the United Kingdom and Scandinavia. Extensive volcanism after the Flood would cause acid rain, which would quickly weather surfaces that were not immediately covered by snow and ice. Therefore, the unique post-Flood climate can explain features like saprolites up to 60 m thick in northeast Scotland which are said to require a warm, humid climate.64,65
Blockfields likely formed on exposed low-relief mountaintops or plateaus as the Ice Age developed. The rare erratics found in some blockfields62,66 indicate that in these cases, the blockfields were likely eventually covered by shallow snow or ice that was not erosive. It is likely that valley ice locally overtopped these blockfields but melted early, causing little mountaintop erosion. Upon deglaciation, meltwater would form rivers and lakes at the top of the ice sheets, as observed today in Greenland. Some of these lakes likely formed along the edge of nunataks because the ice adjacent to nunataks would melt faster due to the lower albedo of the rock. So, erratic boulders caught within the ice can be transported in icebergs by glaciofluvial and glaciolacustrine activity onto the blockfields.
An argument for a thick ice sheet made by secular scientists is that the ice sheets moved too far out onto the shallow continental shelf for them to be thin.66 They claim this is proved by glacial moraines and other features on the ocean bottom. However, a relatively thin ice sheet, such as one that is 500–1,000 m thick at low elevation inland areas, should be able to spread ice far out onto the continental shelf. Several properties of the ice in the biblical model favour rapid motion, especially that post-Flood ice was ‘warm’ with volcanic particles. Together with the thinness of the ice sheet and its relative warmth, the ice would flow significantly faster and farther.87 Given this situation, there would be many surges resulting in the top of the ice becoming low sloped, as has been abundantly deduced at the edge of former ice sheets, such as the Laurentide Ice Sheet in the US Midwest.88
Evidence also points to only one, short Ice Age. It is difficult to conceive of these delicate features surviving even one short Ice Age, while two or more long ice ages would surely erode these features.
Discussion
Evidence has been presented in the form of delicate landscape and soil features that the Scandinavian and British-Irish Ice Sheets were thin. Such features are difficult to explain by 40–50 uniformitarian ice ages of various intensities, but do support one short Ice Age with relatively thin ice cover. Thin ice also means that sea level at glacial maximum was not nearly as low as uniformitarian estimates, which are about –120 m.
Because the post-Flood conditions included volcanism and warm oceans, the ice sheets had some unique features. For instance, the warm oceans would have delayed glacial onset on the western sides of continents. This would include the British-Irish Ice Sheet. This delay was a result of warm, onshore flow. Once the ocean temperatures dropped enough the ice sheet would have grown rapidly. Moreover, the warm air that flowed from the ocean brought very heavy precipitation, which explains why Ice Age hippo fossils are found in north-west Europe. Furthermore, storm tracks would be most common south of the ice sheets. Therefore, it is possible that the ice was relatively thick in the Scandinavian Mountains and along the southern edge of the Scandinavian and Laurentide Ice Sheets, but thinner inland.
A post-Flood Ice Age can also explain the ice domes over Keewatin and the Queen Elizabeth Islands in Canada, as well as the islands and seas north of Norway. In the uniformitarian paradigm, these areas should be polar deserts. However, a warm Arctic Ocean that had no sea ice until the end of the Ice Age would result in an onshore flow of moist air that would build up these ice domes.
The evidence also challenges the 2.6 Ma timescale of the Pleistocene with its 40–50 separate ice ages. Such development and melting of each ice sheet should destroy non-glacial landforms. One ice age with thin ice sheets suggests that the Pleistocene was around 700 years long, not 2.6 Ma.
References and notes
- Bloom, A.L., Glacial-eustatic and isostatic controls of sea level; in: Turekian, K.K. (Ed.), The Late Cenozoic Glacial Ages, Yale University Press, New Haven, CT, p. 367, 1971. Return to text.
- Christoffersen, P., Tulaczyk, S., Wattrus, N.J., Peterson, J., Quintana-Krupinski, N., Clark, C.D., and Sjunneskog, C., Large subglacial lakes beneath the Laurentide Ice Sheet inferred from sedimentary sequences, Geology 36(7):563–566, 2008. Return to text.
- Oard, M.J., Evidence strongly suggests the Laurentide Ice Sheet was thin, J. Creation 30(1):97–104, 2016. Return to text.
- Landvik, J.Y., Brook, E.J., Gualtieri, L., Raisbeck, G., Salvigsen, O., and Yiou, F., Northwest Svalbard during the last glaciation: ice-free areas existed, Geology 31(10):905–908, 2003. Return to text.
- Mangerud, J., Svendsen, J.I., and Astakhov, V.I., Age and extent of the Barents and Kara ice sheets in Northern Russia, Boreas 28:46–80, 1999 Return to text.
- Ottesen, D., Dowdeswell, J.A., and Rise, L., Submarine landforms and the reconstruction of fast-flowing ice streams within a large Quaternary ice sheet: the 2500-km-long Norwegian-Svalbard margin (57°–80°N), GSA Bulletin 117(7/8):1033–1050, 2005. Return to text.
- Astakhov, V.I., Pleistocene glaciations of northern Russia—a modern view, Boreas 42:1–24, 2013. Return to text.
- Andreassen, K., Winsborrow, M.C.M., and Bjarnadóttir, L.R., Ice stream retreat dynamics inferred from an assemblage of landforms in the northern Barents Sea, Quaternary Science Reviews 92:246–257, 2014. Return to text.
- Kleman, J., Stroeven, A.P., and Lundqvist, J., Patterns of Quaternary ice sheet erosion and deposition in Fennoscandia and a theoretical framework for explanation, Geomorphology 97:73–90, 2008. Return to text.
- Fredin, O., Glacial inception and Quaternary mountain glaciations in Fennoscandia, Quaternary International 95–96:99–112, 2002. Return to text.
- Hall, A.M., Sarala, P., and Ebert, K., Late Cenozoic deep weathering patterns on the Fennoscandian shield in northern Finland: a window on ice sheet bed conditions at the onset of Northern Hemisphere glaciation, Geomorphology 246:472–488, 2015. Return to text.
- Stroeven, A.P. et al., Deglaciation of Fennoscandia, Quaternary Science Reviews 147:91–121, 2016. Return to text.
- Oard, M.J., Varves—the first “absolute” chronology, part I: historical development and the question of annual deposition, Creation Research Society Quarterly 29:72–80, 1992. Return to text.
- Oard, M.J., Varves—the first “absolute” chronology, part II: varve correlation and the post-glacial time scale, Creation Research Society Quarterly 29:120–125, 1992. Return to text.
- “A fjord is a long, narrow, winding, glacially-eroded inlet or arm of the sea, U-shaped and steep-walled, generally several hundred meters deep between high rocky cliffs or slopes along a mountainous coast”. Neuendorf, K.K., Mehl, Jr., J.P., and Jackson, J.A., Glossary of Geology, 5th edn, American Geological Institute, Alexandria, VA, p. 240, 2005. Return to text.
- Hättestrand, C. and Stroeven, A.P., A relict landscape in the centre of Fennoscandian glaciation: geomorphological evidence of minimal Quaternary glacial erosion, Geomorphology 44:127–143, 2002. Return to text.
- Greenwood, S.L. and Clark, C.D., The sensitivity of subglacial bedform size and distribution to substrate lithological control, Sedimentary Geology 232:130–144, 2010. Return to text.
- Kleman, J. and Glasser, N.F., The subglacial thermal organization (STO) of ice sheets, Quaternary Science Reviews 26:585–597, 2007. Return to text.
- Hall, A.M., Ebert, K., and Hättestrand, C., Pre-glacial landforms inheritance in a glaciated shield landscape, Geografiska Annaler 95A:33–49, 2012. Return to text.
- Bradwell, T., et al., The northern sector of the last British Ice Sheet: maximum extent and demise, Earth-Science Reviews 88:207–226, 2008. Return to text.
- Sejrup, H.P., Clark, C.D., and Hjelstuen, B.O., Rapid ice sheet retreat triggered by ice stream debuttressing: evidence from the North Sea, Geology 44(5):355–358, 2016. Return to text.
- Barth, A.M., Clark, P.U., Clark, J., McCabe, A.M., and Caffee, M., Last glacial maximum cirque glaciation in Ireland and implications for reconstructions of the Irish Ice Sheet, Quaternary Science Reviews 141: 85–93, 2016. Return to text.
- Stone, J.O. and Ballantyne, C.K., Dimensions and deglacial chronology of the Outer Hebrides Ice Cap, northwest Scotland: implications of cosmic ray exposure dating, J. Quaternary Science 21(1):75–84, 2006. Return to text.
- Dove, D., Arosio, R., Finlayson, A., Bradwell, T., and Howe, J.A., Submarine glacial landforms record Late Pleistocene ice-sheet dynamics, Inner Hebrides, Scotland, Quaternary Science Reviews 123:76–90, 2015. Return to text.
- Krabbendam, M., Eyles, N., Putkinen, N., Bradwell, T., and Arbelaez-Moreno, L., Streamlined hard beds formed by palaeo-ice streams: a review, Sedimentary Geology 338:24–50, 2016. Return to text.
- Neuendorf, K.K., Mehl, Jr., J.P., and Jackson, J.A., Glossary of Geology, 5th edn, American Geological Institute, Alexandria, VA, p. 676, 2005. Return to text.
- Goodfellow, B.W., Relict non-glacial surfaces in formerly glaciated landscapes, Earth-Science Reviews 80:47–73, 2007. Return to text.
- Hättestrand and Stroeven, ref. 16, p. 138. Return to text.
- André, M.-F., The geomorphic impact of glaciers as indicated by tors in North Sweden (Aurivaara, 68° N), Geomorphology 57:403–421, 2004. Return to text.
- Neuendorf et al., ref. 26, p. 574. Return to text.
- Hall, A.M., Sarala, P., and Ebert, K., Late Cenozoic deep weathering patterns on the Fennoscandian shield in northern Finland: a window on ice sheet bed conditions at the onset of Northern Hemisphere glaciation, Geomorphology 246:472–488, 2015. Return to text.
- Stroeven, A.P., Harbor, J., Fabel, D., Kleman, J., Hättestrand, C., Elmore, D., Fink, D., and Fredin, O., Slow, patchy landscape evolution in northern Sweden despite repeated ice-sheet glaciation: in: Willett, S.D., Hovisu, N., Brandon, M.T., and Fisher, D.M. (Eds.), Tectonics, Climate, and Landscape Evolution, GSA Special Paper 398, Geological Society of America, Boulder, CO., pp. 387–396, 2006. Return to text.
- Neuendorf et al., ref. 26, p. 73. Return to text.
- Goodfellow, B.W., Fedin, O., Derron, M.-H., and Stroeven, A.P., Weathering processes and Quaternary origin of an alpine blockfield in Arctic Sweden, Boreas 38:379–398, 2008. Return to text.
- Ballantyne, C.K. and Stone, J.O., Trimlines, blockfields, and the vertical extent of the last ice sheet in southern Ireland, Boreas 44:277–287, 2015. Return to text.
- Paasche, Ø, Raukleiv, J., Dahl, S.O., and Linge, H., Weathering characteristics of arctic islands in northern Norway, Geomorphology 82:430–452, 2006. Return to text.
- Rea, B.R., Whalley, W.B., Rainey, M.M., and Gordon, J.E., Blockfields, old or new? Evidence and implications from some plateaus in northern Norway, Geomorphology 15:109–121, 1996. Return to text.
- Ballantyne, C.K., A general model of autochthonous blockfield evolution, Permafrost and Periglacial Processes 21:289–300, 2010. Return to text.
- Goodfellow, B.W., A granulometry and secondary mineral fingerprint of chemical weathering in periglacial landscapes and its application to blockfield origins, Quaternary Science Reviews 57:121–135, 2012. Return to text.
- Goodfellow, B.W., Stroeven, A.P., Fabel, D., Fredin, O., Derron, M.-H., Bintanja, R., and Caffee, M.W., Arctic-alpine blockfields in the northern Swedish Scandes: late Quaternary—not Neogene, Earth Surface Dynamics 2:383–401, 2014. Return to text.
- Hopkinson, C. and Ballantyne, C.K., Age and origin of blockfields on Scottish mountains, Scottish Geographical J. 130(2):116–141, 2014. Return to text.
- Goodfellow et al., ref. 40, p. 383. Return to text.
- Neuendorf et al., ref. 26, p. 445. Return to text.
- Hall, A.M. and Sugden, D.E., Limited modification of mid-latitude landscapes by ice sheets: the case of northeast Scotland, Earth Surface Processes and Landforms 12:531–542, 1987. Return to text.
- Kleman, J., Preservation of landforms under ice sheets and ice caps, Geomorphology 9:19–32, 1994. Return to text.
- Gilg, H.A., Hall, A.M., Ebert, K., and Fallick, A.E., Cool kaolins in Finland, Palaeogeography, Palaeoclimatology, Palaeoecology 392:454–462, 2013. Return to text.
- Darmody, R.G., Thorn, C.E., Seppälä, M., Campbell, S.W., Li, Y.K., and Harbor, J., Age and weathering status of granite tors in Arctic Finland (~68°N), Geomorphology 94:10–23, 2008. Return to text.
- Ebert, K. and Hättestrand, C., The impact of quaternary glaciations on inselbergs in northern Sweden, Geomorphology 115:56–66, 2010. Return to text.
- Ebert, K., Hall, A.M., Kleman, J., and Andersson, J., Unequal ice-sheet erosional impacts across low-relief shield terrain in northern Fennoscandia, Geomorphology 233:64–74, 2015. Return to text.
- Sarapää, O. and Sarala, P., Rare earth element and gold exploration in glaciated terrain: example from the Mäkärä area, northern Finland, Geochemistry: Exploration, Environment, Analysis 13:131–143, 2013. Return to text.
- Stroeven, A.P., Fabel, D., Hättestrand, C., and Harbor, J., A relict landscape in the centre of Fennoscandian glaciation: cosmogenic radionuclide evidence of tors preserved through multiple glacial cycles, Geomorphology 44:145, 2002. Return to text.
- Hall, et al., ref. 11, p. 473. Return to text.
- White, W.A., Deep erosion by continental ice sheets, GSA Bulletin 83:1037–1056, 1972. Return to text.
- White, W.A., More on deep glacial erosion by continental ice sheets and their tongues of distributary ice, Quaternary Research 30:137–150, 1988. Return to text.
- Lundqvist, J., The Revsund area, central Jämtland—an example of pre-glacial weathering and landscape formation, Geografiska Annaler 70A:291–298, 1988. Return to text.
- Sugden, D.E., A case against deep erosion of shields by ice sheets, Geology 4:580–582, 1976. Return to text.
- Ebert, K., Hall, A.M., and Hättestrand, C., Pre-glacial landforms on a glaciated shield: the inselberg plains of northern Sweden, Norwegian J. Geology 92:1–17, 2012. Return to text.
- Lidmar-Bergström, K., Ollier, C.D., and Sulebak, J.R., Landforms and uplift history of southern Norway, Global and Planetary Change 24:211–231, 2000. Return to text.
- Hall, A.M., Ebert, K., Kleman, J., Nesje, A., and Ottesen, D., Selective glacial erosion of the Norwegian passive margin, Geology 41(12):1203–1206, 2013. Return to text.
- Lidmar-Bergström, K., A long-term perspective on glacial erosion, Earth Surface Processes and Landforms 22:297–306, 1997. Return to text.
- Hall, A.M. and Mellor, A., The characteristics and significance of deep weathering in the Gaick area, Grampian Highlands, Scotland, Geografiska Annaler 70A:309–314, 1988. Return to text.
- Clark, C.D., Evans, D.J.A., Khatwa, A., Bradwell, T., Jordan, C.J., Marsh, S.H., Mitchell, W.A., and Bateman, M.D., Map and GIS database of glacial landforms and features related to the last British Ice Sheet, Boreas 33:359–375, 2004. Return to text.
- Ballantyne, C.K., Extent and deglacial chronology of the last British-Irish Ice Sheet: implications of exposure dating using cosmogenic isotopes, J. Quaternary Science 25(4):515–534, 2010. Return to text.
- Hall, A.M., Cenozoic weathering covers in Buchan, Scotland, and their significance, Nature 315:392–395, 1985. Return to text.
- Hall, A.M., Deep weathering patterns in north-east Scotland and their geomorphological significance, Zeitschrift für Geomorphologie N.F. 30(4):407–422, 1986. Return to text.
- Fabel, D., Ballantyne, C.K., and Xu, S., Trimlines, blockfields, mountain-top erratics and the vertical dimensions of the last British-Irish Ice Sheet in NW Scotland, Quaternary Science Reviews 55:91–102, 2012. Return to text.
- Hall, A.M. and Phillips, W.M., Glacial modification of granite tors in the Cairngorms, Scotland, J. Quaternary Science 21(8):811–830, 2006. Return to text.
- Finlayson, A., Fabel, D., Bradwell, T., and Sugden, D., Growth and decay of a marine terminating sector of the last British-Irish Ice Sheet: a geomorphological reconstruction, Quaternary Science Reviews 83:28–45, 2014. Return to text.
- Mitchell, W.A., Quaternary geology of part of the Kale Water catchment, Western Cheviot Hills, southern Scotland, Scottish J. Geology 44(1):51–63, 2008. Return to text.
- Smith, A., The Ice Age in the Lake District, Rigg Side Publications, Grange Park, Keswick, Cumbria, UK, 2008. Return to text.
- Battiau-Queney, Y., The pre-glacial evolution of Wales, Earth Surface Processes and Landforms 9:229–252, 1984. Return to text.
- Ollier, C., Ancient Landforms, Belhaven Press, New York, p. 205, 1991. Return to text.
- Smith, B.J. and McAlister, J.J., Tertiary weathering environments and products in northeast Ireland; in: Gardiner, V. (Ed.), International Geomorphology 1986, Part II, John Wiley & Sons Ltd, New York, pp. 1,007–1,031, 1987. Return to text.
- Walker, M. and Lowe, J., Quaternary science 2007: a 50-year retrospective, J. the Geological Society London 164:1073–1092, 2007. Return to text.
- Kleman, J. and Glasser, N.F., The subglacial thermal organization (STO) of ice sheets, Quaternary Science Reviews 26:585–597, 2007. Return to text.
- Hebert, J., Circular reasoning in the dating of deep seafloor sediments and ice cores: the orbital tuning method, Answers Research J. 7:297–309, 2014. Return to text.
- Hebert, J., Should the “pacemaker of the ice ages” paper be retracted? Revisiting an iconic argument for Milankovitch climate forcing, part 1, Answers Research J. 9:25–56, 2016. Return to text.
- Hebert, J., Revisiting an iconic argument for Milankovitch climate forcing: should the “pacemaker of the ice ages” paper be retracted? part 2, Answers Research J. 9:131–147, 2016. Return to text.
- Reed, J.K. and Oard, M.J., Cyclostratigraphy, part I: What is cyclostratigraphy? Creation Research Society Quarterly 52(2):95–105, 2015. Return to text.
- Ebert, K., Terminology of long-term geomorphology: a Scandinavian perspective, Progress in Physical Geography 33(2):163–182, 2009. Return to text.
- Margold, M., Stokes, C.R., and Clark, C.D., Ice streams in the Laurentide Ice Sheet: identification, characteristics and comparison to modern ice sheets, Earth-Science Reviews 143:117–146, 2015. Return to text.
- Ebert, ref. 80, p. 163. Return to text.
- Oard, M.J., Frozen in Time: Woolly mammoths, the Ice Age, and the biblical key to their secrets, Master Books, Green Forest, AR, 2004. Return to text.
- Oard, M.J. (DVD), The Great Ice Age: Evidence from the Flood for Its Quick Formation and Melting, Awesome Science Media, Canby, OR, 2013. Return to text.
- Oard, M.J., Flood by Design: Receding water shapes the earth’s surface, Master Books, Green Forest, AR, 2008. Return to text.
- Oard, M.J. (ebook), Earth’s Surface Shaped by Genesis Flood Runoff, 2013, Michael.oards.net/GenesisFloodRunoff.htm. Return to text.
- Paterson, W.S.B., The Physics of Glaciers, 2nd edn, Pergamon Press, New York, 1981. Return to text.
- Oard, M.J., Evidence strongly suggests the Laurentide Ice Sheet was thin, J. Creation 30(1):97–104, 2016. Return to text.
- Oard, M.J. and Oard, B., Life in the Great Ice Age, Master Books, Green Forest, AR, 1993. Return to text.
- Hughes, A.L.C., Clark, C.D., and Jordan, C.J., Flow-pattern evolution of the last British Ice Sheet, Quaternary Science Reviews 89:148–168, 2014. Return to text.
Readers’ comments
Comments are automatically closed 14 days after publication.