Journal of Creation 27(1):16–18, April 2013
Browse our latest digital issue Subscribe
Internal oceanic waves and sedimentation
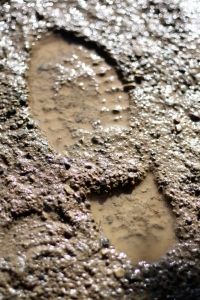
It is so easy for critics of the Genesis Flood, such as evolutionary/uniformitarian geologists and old-earth Christians, to come up with supposed contradictions upon a superficial analysis and lack of information. One of those instances was the deposition of mud, believed to settle very slowly. With over two thirds of sedimentary rocks being mudstones or shales, the deposition of mud was considered to take too much time for the one-year Flood. However, this superficial deduction was recently turned on its head with the discovery that mud can be deposited rapidly even in a current.1–5
Rapid mud deposition
More details on the rapid deposition of mud are coming out with more flume experiments and the analysis of mud in the oceans. Researchers have recognized that they do not understand the full range of variables in mud dispersal and accumulation. In fact, mud deposition is considered so complex that it may take as many as 32 variables to understand mud sediments.6
Although an individual mud particle settles very slowly, it has been discovered that mud particles commonly coagulate or flocculate due to electrostatic forces. The larger the floccules (or flocs—the individual aggregates of the mud), the faster they settle.7 With a little turbulence (but not too much as that would break up the floccule) individual particles come together and floccules become larger.8 Floccule size increases with higher organic content, higher salt content, and lower pH.9 Flocs have even been observed that can settle around 100 to 200 m/day.
Rapid transport of mud on the continental shelf
Physical oceanographers have concluded that currents cannot transport mud across the continental shelf into deeper water because coastal currents are predominantly parallel to bathymetry. Moreover, the seaward gradient of the bathymetry is too low for transport by gravity-driven (mass flow) processes. Nevertheless, mud has been observed being transported across the shelf.10 Several proposed solutions appear to have shortcomings. Then how is mud transported?
In attempting to answer this question, scientists have discovered a new transport mechanism:
“A major recent discovery in marine sedimentology is a new class of gravity-driven sediment flows, referred to as wave-enhanced sediment-gravity flows (WESGFs), wherein the energy to maintain sediment in suspension is provided by orbital motion of surface gravity waves, instead of gravity-induced turbulence, as for classical turbidity currents.”11
In other words, the waves on the sea surface, especially during storms, induce waves in shallow water at the mud/water density interface that propagate downslope, even on a very low bathymetric gradient. The wave causes mud suspension that is transported as a mass flow with the wave and deposited farther downslope.
The resulting deposits of these WESGFs have been cored along the Eel River continental shelf off northern California, USA. Similar results have also been observed off the Po River of northeast Italy.12 The WESGFs result in multiple thin layers. The basal contact of each WESGF is erosional with a relief of 3 mm. The bottom lamina is composed of silt-sized sediment of quartz and clay that is either homogeneous or possesses low-angle cross beds. Above the basal lamina, there are multiple lamina sets composed of intercalated clay-rich and quartz-rich sediments caused by laminar (non-turbulent) flow.
Researchers have looked at mudstones and shales in sedimentary rock and are now discovering the same features observed in WESGF deposits, e.g. the large-scale ‘Cretaceous’ Mowry Shale of the High Plains and Rocky Mountains of the United States. This suggests that deposition of the Mowry Shale was rapid:
“Their presence in the Mowry Shale suggests that wave reworking and advective [horizontal] sediment transport processes operated to control lithofacies variability in this succession, so this unit cannot have been deposited in a persistently low-energy basin, contrary to existing models.”13
Macquaker et al. go on to conclude:
“The presence of these diagnostic fabrics, distinct from classical turbidites and tempestites [storm deposits] as well as other fluid-mud deposits, calls for a reappraisal of other ancient mudstone successions deposited on continental shelves, as their presence will revolutionize geological interpretations of the physical processes responsible for their accumulation.”14
Internal waves can also cause WESGFs
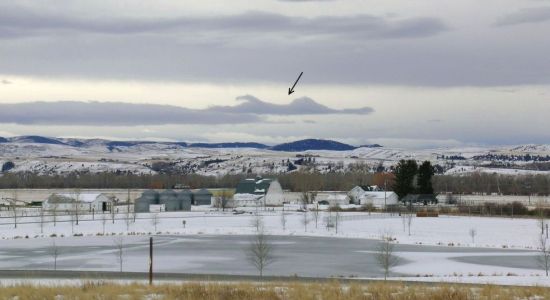
In the research reported above, WESGFs were caused by surface waves, especially from storms. However, it is now known that internal waves can also cause WESGFs. Internal waves are subsurface-propagating gravity waves caused by a perturbation on a pycnocline, a boundary between two density-stratified fluids or a layer with a vertical density gradient.15 These waves are similar to gravity or Kelvin-Helmholtz waves in the atmosphere (figure 1).16 A pycnocline can include a mud/water interface, the thermocline, an interface between a river plume and clear water, or a salinity gradient. Such internal waves have wavelengths up to 350 km and displace water parcels over 100 m. Internal waves are ubiquitous in the oceans today, being more common than surface waves! They also occur in lakes.
The perturbation that causes internal waves can be the result of surface waves generated by storms, such as observed with WESGFs, or from a current flowing over an underwater barrier.17 Internal waves have been observed by satellite at the boundary of river plumes,18 along the Sri Lanka continental slope due to a tsunami,19 and in the western Indian Ocean caused by a gap in the Mascarene undersea plateau. The tsunami that hit Sri Lanka in December of 2004 caused internal waves that were reflected, diffracted, and scattered from the continental slope, even causing the brief suspension of sand. Waves were observed to travel about 3 m/sec both to the west and east of the Mascarene Plateau of the western Indian Ocean caused by westerly currents. The eastward-propagating waves were weaker and caused by reflection from the plateau. Internal waves cause sediment erosion, transport, and re-deposition, even in the deep ocean.20 Internal waves are believed to have significantly impacted the sedimentary record, although sedimentologists have been unaware of them:
“Internal waves are waves that propagate along the interface between two different density fluids (pycnocline). Since internal waves are very common in oceans and lakes, their effects on sediments should be common too. However, they have remained largely unrecognized by sedimentologists; they have previously been ascribed to being the products of storms and/ or turbid currents, or may have been simply identified as deposits of some unexplained phenomena.”21
It is interesting how crucial observations can be missed and can potentially result in a paradigm shift in a field of study, like sedimentology.
Application to Flood geology
Project the above information on internal waves and WESGFs to the Genesis Flood, which had orders of magnitude more sediment available, much stronger currents at times, multiple tsunamis, huge ocean waves, a large amount of organic matter to cause larger flocs, and multiple underwater barriers. Of course flocs would not form in highly turbulent floodwater, which would be expected early in the Flood, but as the Flood progressed and the currents and turbulence slowed, flocs would more easily form and sedimentation would be rapid.
In addition, there would be abundant pycnoclines, especially at the sediment/water interface, during rapidly accumulating sediments. Other pycnoclines would occur in strong salinity gradients between floodwater, rainwater, and continental runoff and ocean water (which may not have been as salty as today). Strong temperature gradients caused by volcanism, the fountains of the great deep, and other factors would cause numerous internal waves. The surface waves and internal waves would go wild throughout the Flood with variable strengths, velocities, sediment, and sediment transport. With the stronger internal waves in the Flood compared to today, sand would commonly be transported, as well as mud. Moreover, WESGFs would not be confined to shallow water, as observed today, but can occur in water of any depth. It is well known that internal waves stir up and redeposit sediments in the deep ocean today.
Such internal waves can potentially explain many features observed in sedimentary rocks. They can explain multiple bedding planes that are sharp, since the bases of WESGFs are mostly erosional with little relief. Internal waves can explain multiple, thin layers, such as between silt and clay or sand and mud couplets22 that are often interpreted as varves, a rhythmite of one year deposition. Many silt/clay, sand/mud, or any other types of oscillating rhythmites can be deposited very rapidly in one internal wave, but with a huge number of internal waves, tens of thousands of couplets can potentially be deposited in a short time. Youbin et al. state:
“The sedimentary microfacies types of the identified internal-wave and internal-tide deposits are summarized, such as bidirectional cross-lamination sandstone microfacies, unidirectional crossbedding and cross-lamination sandstone microfacies, rhythmic alternating thin sandstone and mudstone microfacies … . Rhythmic alternating thin sandstone and mudstone microfacies were found in all the documented internal-wave and internal deposits in China … . They are characterized by frequently alternating beds, consisting of thin sandstone and thin mudstone.”23
Such thin layers are abundant in the sedimentary rock record, for instance the 6.5 million couplets in the Green River Formation used to suggest millions of years of deposition.24
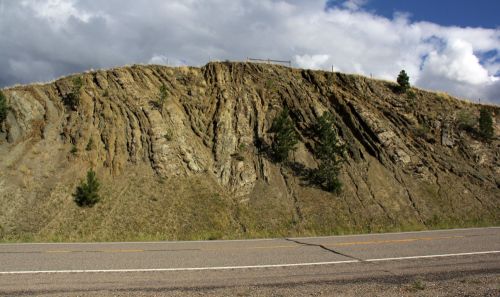
Internal waves are capable of laying down thicker rhythmites such as common sand/shale couplets seen in sedimentary rocks. It is not a stretch to extrapolate with the thin layers laid down today by WESGFs on a scale of a few cm to sand/shale couplets on a metre scale. Figure 2 shows alternating coarse-grained and fine grained sedimentary rocks in a chevron fold.
Rhythmites may have regular features or sedimentary layers in ‘packages’ showing several scales of oscillations by internal waves, for instance a coarse-/fine-grained couplet every 10 cm within a thicker layering of 2 m. Such packages are relatively common in the sedimentary rocks and recently have been claimed to be Milankovich oscillations caused by cycles in the earth’s orbital geometry. These sedimentary packages are now used extensively to date sedimentary rocks to a ‘very fine’ resolution of 22,000 years (22 ka) within the evolutionary/uniformitarian timescale (the 22 ka cycle is the precessional cycle). This dating scheme is called cyclostratigraphy and is commonly used to ‘date’ sedimentary rocks to 50 Ma and beyond.25
Internal waves may be able to explain the data of sequence stratigraphy, ‘transgression/regression events’, and other features of the sedimentary rocks that are used either to date rocks or to support ‘deep time’. Supposed transgressive/regressive sequences can be simply explained by waning and waxing current velocity, either from an internal wave or a Flood current.
References
- Walker, T., Mud experiments overturn long-held geological beliefs, J. Creation 22(2):14–15, 2008. Return to text.
- Schieber, J., Southard, J. and Thaisen, K., Accretion of mudstone beds from migrating floccule ripples, Science 318:1760–1763, 2007. Return to text.
- Macquaker, J.H.S. and Bohacs, K.M., On the accumulation of mud, Science 318:1734–1735, 2007. Return to text.
- Austin, S.A., Understanding the mudrock revolution and the global Flood as described in Scripture, Sixth International Conference on Creationism in Pittsburgh, PA, 4 August 2008. Return to text.
- Morris, J.D., The Global Flood: Unlocking Earth’s Geologic History, Institute for Creation Research, Dallas, TX, p. 113, 2012. Return to text.
- Schieber et al., ref. 2, p. 1760. Return to text.
- Strom, K. and Keyvani, A., An explicit full-range settling velocity equation for mud flocs, J. Sedimentary Research 81:921–934, 2011. Return to text.
- Kumar, R.G., Strom, K.B. and Keyvani, A., Floc properties and settling velocity of San Jacinto estuary mud under variable shear and salinity conditions, Continental Shelf Research 30:2067–2081, 2010. Return to text.
- Mietta, F., Chassagne, C., Manning, A.J. and Winterwerp, J.C., Influence of shear rate, organic content, pH and salinity on mud flocculation, Ocean Dynamics 59:751–763, 2009. Return to text.
- Macquaker, J.H.S., Bentley, S.J. and Bohacs, K.M., Wave-enhanced sediment-gravity flows and mud dispersal across continental shelves: reappraising sediment transport processes operating in ancient mudstone successions, Geology 38:947–950, 2010. Return to text.
- Macquaker et al., ref. 10, p. 947. Return to text.
- Trayovski, P., Wiberg, P.L. and Geyer, W.R., Observations and modeling of wave-supported sediment gravity flows on the Po prodelta and comparison to prior observations from the Eel shelf, Continental Shelf Research 27:375–399, 2007. Return to text.
- Macquaker et al., ref. 10, p. 949. Return to text.
- Macquaker et al., ref. 10, p. 950. Return to text.
- Pomar, L., Morsilli, M., Hallock, P. and Bádenas, B., Internal waves, an under-explored source of turbulence events in the sedimentary record, Earth-Science Reviews 111:56–81, 2012. Return to text.
- Oard, M.J., Application of a diagnostic Richardson number equation to a case study of clear air turbulence, J. Applied Meteorology 13(7):771–777, 1974. Return to text.
- Da Silva, J.C.B., New, A.L. and Magalhaes, J.M., On the structure and propagation of internal solitary waves generated at the Mascarene Plateau in the Indian Ocean, Deep-Sea Research I 58:229–240, 2011. Return to text.
- Nash, J.D. and Moum, J.N., River plumes as a source of large-amplitude internal waves in the coastal ocean, Nature 437:400–403, 2005. Return to text.
- Santek, D.A. and Winguth, A., A satellite view of internal waves induced by the Indian Ocean tsunami, International J. Remote Sensing 28:2927–2936, 2007. Return to text.
- Youbin, H., Zhenzhong, G., Jinxiong, L., Shunshe, L. and Xuefeng, L., Characteristics of internal-wave and internal-tide deposits and their hydrocarbon potential, Petroleum Science 5:37–44, 2008. Return to text.
- Pomar et al., ref. 15, p. 78. Return to text.
- Pomar et al., ref. 15, p. 66. Return to text.
- Youbin et al., ref. 20, p. 39. Return to text.
- Oard, M.J. and Klevberg, P., Green River Formation very likely did not form in a postdiluvial lake, Answers Research J. 1:93–107, 2008. Return to text.
- Hinnov, L.A. and Hilgen, F.J., Cyclostratigraphy and astrochronology; in: Gradstein, (Eds.), F.M., Ogg, J.G., Schmitz, M.D. and Ogg, G.M., The Geological Time Scale 2012, vol. 1, Elsevier, New York, pp. 63–83, 2012. Return to text.
Readers’ comments
Comments are automatically closed 14 days after publication.